Localised renewable energy is an affordable, clean alternative to fossil fuels that is gaining popularity for use in remote ‘off-grid’ communities [1]-[4], particularly in low and lower-middle income countries [5], where reducing costs make it more feasible [4], [6], [7]. To support such communities and their ecosystems, national and international organizations often provide development assistance programs alongside specific forms of humanitarian intervention. Key goals of these programs, respectively, include enhancing community capabilities regarding energy sufficiency [8]-[10] and disaster risk reduction at the local level [11]-[13].
Access to resources and knowledge on how to establish, operate, repair and maintain local infrastructure and services increases community self-sufficiency. Frequently however, this is not the case for the remote, riparian communities in low and lower middle-income countries. Assistance provided to these communities is often temporary, or promised longer-term solutions fail over time as they cannot support (or be supported by) end-users in a realistic and sustainable manner. Such communities are often small, agriculture-based monoeconomies with low incomes, power insufficiencies, and increased exposure to extreme weather events due, in part, to limited response capability and technological support [14]-[16]. In this study, communities with such characteristics are described as vulnerable or remote. Its focus is on their current use of support technologies and potential future development of such assets.
The United Nations 2030 Agenda for Sustainable Development [8] highlights the importance of Sustainable Development Goal 7: Affordable and Clean Energy as essential for socio-economic development and self-sufficiency, as well as for the success of other Goals [17]. Off-grid renewable energy systems (OGRES), such as small-scale hydropower generators and solar panels, offer a range of potential benefits to vulnerable communities, including enhanced disaster response capability when generated power can support flood early-warning systems (EWS) [18]. According to the Sendai Framework for Disaster Risk Reduction, community resilience against natural hazards is an essential element of sustainable development [11]. Successfully combining OGRES and EWS would provide a comprehensive humanitarian engineering solution that accords with the principles of both of these international frameworks [19].
In this study, humanitarian engineering is defined as the technology-focused applications that can support the basic needs (e.g. energy generation, shelter, water sanitation) of an affected population, including preparation for, and response to hazard events [20]-[22]. Such applications can be either temporary or permanent [23]. Humanitarian engineering is often conceived by aid practitioners and field engineers as providing innovation and ‘elegant solutions’ to more than one issue simultaneously (e.g. a micro-hydropower system for irrigation may also power public lights and a school facility) [24], [25]. While humanitarian engineering has the potential to provide such multi-faceted solutions in vulnerable communities, a key requirement is that such solutions are sustainable over time and within community resources, especially after the professionals leave or external funding discontinues.
To evaluate sustainability, it is essential to determine the appropriateness of a proposed technology to the community (perceived value and sustained use) and its environmental context (i.e. low and/or positive impact). So-called ‘appropriate technology’ frameworks consider local characteristics and allow slow adaptations while end-user communities reach and maintain sustainable forms of development [26]-[29]. Assessments of technology appropriateness for local-level renewable energy systems typically examine technical, economic and social indicators that would support effective use in a specific community [30], [31]. Bauer and Brown (2014) [32] developed the ‘Appropriate Technology Assessment Tool’ which uses 47 indicators to determine technology appropriateness. The tool has a strong evidence base, with its indicators derived from a meta-analysis of 53 articles, books and conference proceedings in appropriate technology. In addition to appropriateness, Ilskog (2008) [33] has argued for additional analysis of the sustainability of community-level technology developments across two categories: environmental and institutional. Her proposed method assesses these across 39 indicators, with these articulating closely with the United Nations Millennium Development Goals, and the United Nations Sustainable Development Goals. Importantly, this assessment framework has also demonstrated specific utility in sustainability evaluations of energy-related projects [34]. The frameworks of Bauer and Brown (2014) [32] and Ilskog (2008) [33] provide comprehensive coverage of the appropriateness and sustainability of community developments which have a technology focus, and both will be used in the evaluation of case studies identified in this review.
This scoping review investigates the uses of OGRES and flood EWS in vulnerable communities, including specific indicators of strengthened or undermined end-user confidence in these systems or their sustainability. While individual case studies have detailed the successes and failures of OGRES or EWS in remote communities, a comparative analysis of these systems following the four-stage framework of Arksey and O’Malley (2005) [35] has not previously been conducted. Such an analysis can inform researchers, government authorities, development, and humanitarian agencies, as well as communities themselves, as to the viability and cost-benefit of such systems in these contexts. These data will also inform a wider study at Western Sydney University in Australia which is examining whether community-level renewable energy sources can reliably power flood EWS as a hybrid system. To the authors’ knowledge, there has been no such study of combined systems for sustainable development and flood resilience at the local level. While some researchers have examined solar energy use in EWS [36]-[38], there are no published data regarding other OGRES (i.e. hydropower) powering localised EWS for community response. While the primary focus of this review is the community use and effectiveness of OGRES and EWS as individual ‘sub’ systems, a secondary focus will be the implications of these findings and potential applications for a future hybrid system. Community-focused features that enhance acceptability and sustainability of the sub-systems among different populations facing similar threats will likely be integral to hybrid systems [39].
This article presents a scoping review analysis that follows the four-stage framework of Arksey and O’Malley (2005) [35]: (i) identification of research questions, (ii) identification of relevant studies, (iii) selection of studies, and (iv) collation and report of findings. This method has been used by many scholars, and has been adopted for use in a wide range of disciplines [40]; for example water, sanitation and hygiene (WASH) [41], aging studies [42], [43], education [44], and occupational safety [45].
The aim of this scoping review is to collect research findings (success cases, lessons and failure factors) related to community-based renewables and flood EWS installed in low and lower middle-income countries. The review was guided by the following questions:
What are the elements of successful OGRES and EWS within vulnerable communities experiencing energy insufficiency and/or flood risk?
What factors contribute to (or enable/disenable) the successful establishment and ongoing maintenance of such systems in low and lower middle-income countries in order to achieve sustainable development?
What features should a hybrid system have in order to be sustainable longer-term?
A range of terms are used in the literature to describe the focus areas highlighted in this article. For example, “renewable energy” is sometimes used interchangeably with terms like “green energy”, “clean energy” or “sustainable energy”. We conducted our search based on the most commonly used version of these terms. The following string was used for the identification of relevant studies: (“success” OR “lesson” OR “failure”) AND (“renewable energy” OR “flood early warning” OR “technology acceptance” OR “technology transfer”) AND (“developing countr*” OR “low income countr*”)
ScienceDirect was selected as the primary source for research articles in multi-disciplinary themes (e.g. engineering, energy, environmental, social and sustainability) fields. Google Scholar was also used to broadly search for relative scholarly literature. In addition, the following key academic journals were selected for this search due to their high relevance in their fields:
Energy for Sustainable Development
Engineering Failure Analysis
Environmental Science & Policy
Journal of Cleaner Production
Journal of Rural Studies
International Journal of Disaster Risk Reduction
Procedia Engineering
Progress in Disaster Science
Renewable Energy
Renewable and Sustainable Energy Reviews
Lastly, the authors examined so-called ‘grey literature’, which is understood as literature produced and/or delivered by international and multinational organizations and agencies that are not primarily focus on ‘traditional’/academic publishing. The following bodies were searched:
International Centre for Integrated Mountain Development
International Renewable Energy Agency
United Nations Framework Convention on Climate Change
United Nations University Institute of Environment and Human Security
Review articles, research articles, case reports and product reviews available in English were included; all other types of publications and languages were excluded. As interest in renewable energy and early warning technologies dates roughly from the beginning the selected period was from January 2000 to December 2020. This is a period that has witnessed a plethora of innovations and optimizations. Furthermore, the Sendai Framework for Disaster Risk Reduction, and the 2030 Agenda for Sustainable Development were also initiated during this period, guiding responses for disaster resilience, environmental protection, humanitarian aid and sustainable development.
A total of 2,449 studies were identified from ScienceDirect and Google Scholar, with the period of examination lasting up until December 31st, 2020. After journal searching, 325 studies were retrieved. Firstly, titles and abstracts were screened which resulted in 42 studies. Full-text screening of the included studies resulted in nine studies. After bibliographical searching, one study [14] was additionally included. Two other studies [46], [47] were added due their high relevance to the topic and response to research questions; these were found by using the string keywords in the Google search engine. The ‘grey literature’ search resulted in six reports, while full-text screening led to the inclusion of only two reports. The full-text analysis thus resulted in 14 studies for final inclusion. The entire process is visually explained in Figure 1 and a summary of the studies is provided in Table 1 (Appendix).
The selected-for-inclusion studies were coded following the 6-step process as detailed by Braun and Clarke (2006) [48]. This process includes: (i) data familiarization by reading and re-reading the studies, (ii) initial codes generation; (iii) codes grouping into relevant themes; (iv) review themes to develop a ‘thematic map’, (v) themes definition and naming, and (vi) themes narration.
Study selection flow chart
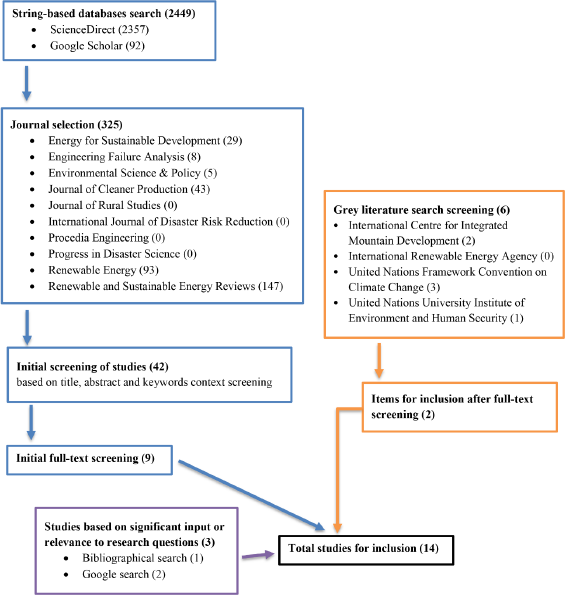
The scoping review led to three major findings:
Despite the differences in socio-cultural, economic and environmental conditions, many communities have similar needs and lack similar capabilities
Many cases shared either the same or similar system failure/success indicators
Long-term community engagement and equitable socio-economic benefits delivery were crucial in the success of both systems, while lack of support, technical know-how, funding and system service were major indicators for failure
Three primary themes were distilled from the findings:
Recognition of diversities and similarities in vulnerable communities
Appropriate technology and sustainability mapping
Enablers for success and failure factors
The review summarised OGRES case studies (hydro, solar, and wind-power, and mixed projects) from Bolivia, Philippines, Nepal, Rwanda, Ethiopia, Gabon, Ghana, Kenya, Malawi, Mozambique, Nigeria, Tanzania, Cameroon, Central African Republic, Chad, Republic of Congo, Afghanistan, and Papua New Guinea. The EWS case studies (flood or multi-hazard) were located in Kenya, Sri Lanka, Egypt, Mali, Afghanistan, India, Nepal, and Pakistan. The findings indicate that most cases for renewables were located in the African region, while for flood EWS they were located in both African and Asian regions. Another observation from the data in Table 1 is that some of the OGRES and flood EWS cases were located in the same country (e.g. Kenya, Nepal). This shows that some regions in these countries are in need of both energy and flood resilience aid.
Besides the similarities in the lack of sufficient energy and flood resilience infrastructure, communities demonstrated differences to each other due to their differing needs, capabilities and socio-cultural makeups. For instance, communities in Bolivia used electricity for powering refrigerators, while communities in the Philippines did not. The OGRES in Bolivia and the Philippines increased income and created more jobs but not in Afghanistan. In Mali, neighboured communities and different groups were in conflict with each other due to water scarcity, however, in Hindu Kush Himalaya, communities worked well together to face floods.
The complimentary assessment frameworks [32], [33] described in Introduction are suitable to combined use to support a comprehensive review of the identified studies. Table 2 (Appendix) summarises the findings and classifies the cases based on information of failure or success outcomes. The following session further details the results of Table 2.
Table 2 presents important information for the technology appropriateness of both OGRES and EWS installations. Importantly, it frames enablers for successful approaches and problem solving, as well as conditions that lead to failure.
Regarding the institutional indicators, community engagement was perhaps the most important enabler for technology appropriateness as it was highlighted in many OGRES and EWS cases. Ownership and management, as well as autonomy were also important, especially when local stakeholders were involved in system development. None of the reviewed OGRES and EWS were reported to have caused any significant impacts to local ecosystems. Conversely, some OGRES were found to have supported ‘damaged’ ecosystems (e.g. reduced use of firewood). Both types of systems were most successful when adjusted to local environmental conditions (e.g. could operate/multi-function in high and low water levels). In social/ethical matters, post-installation improvements in daily activities, health conditions (e.g. reduced use of kerosene) and services, education, socializing and entertainment were crucial enablers for the success and acceptability of both system types. In the economic realm, the generation of income, creation of new jobs and low installation and maintenance costs were major success indicators. With respect to technical matters, and for both systems types, adaptability, simplicity, effectiveness, multi-purposing capacity, and materials availability were good enablers. Notably, one EWS presented hybrid characteristics (self-powered by solar panels).
In terms of failure factors in institutional matters, issues occurred when the legislation and regulations were not appropriate to such systems development. The lack of support of all kinds was also a significant disenabling factor. With respect to the environment, the systems could not operate well when they were not designed for local site conditions, while for social and ethical issues, the lack of benefits or inequitable delivering, fear of identity loss and conflicts, theft and jealously were serious failure factors. Knowledge, training and ease of use were also important criteria for the success or failure of a system. In terms of economics, the most important factor was the high cost of the systems. Furthermore, marketing strategies in local populations also contributed to the acceptance of or apathy toward systems. Lastly, in technical matters, most failures occurred when the system was not appropriately constructed, vulnerable to extreme weather conditions, energy inefficient, poorly maintained, broken, and complex. These issues were primarily the outcomes of a lack of funding, knowledge and skills and overall support.
The findings from this scoping review can help improve humanitarian engineering interventions regarding the appropriateness of OGRES and EWS in remote communities, with the outcome data examining both failure and success factors.
As noted above, OGRES were largely situated in African regions where instability in electrical power provision is common. Africa has the physical characteristics required to develop a range of renewable energy applications and increase energy supply [49]-[52]. For example, the Sub-Saharan region is one of the least developed regions globally with major electrification problems, so there is great potential for OGRES [16], [53]-[60]. The local atmospheric and hydrogeomorphological conditions permit both hydro and solar-power installations. However, countries in the Sub-Saharan region often lack resources, infrastructure, political will and funding to establish such systems on a sustainable basis [50], [54], [61]-[68]. Figure 2 presents the Population without Access to Electricity 2019 Map , developed by the International Energy Agency - IEA [69]. The map focuses on countries in the ‘Global South’.
Population without Access to Electricity 2019 Map.
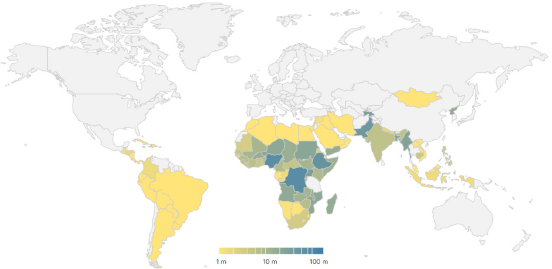
Figure 2 illustrates the serious energy insufficiency of Sub-Saharan Africa. It also shows that regions in Asia (primarily Southeast Asia and Hindu Kush Himalayan) and Latin America are also in need of OGRES as their power capacity is not sufficient. Our review findings articulate with this data.
Besides the Sub-Saharan region, the conditions for renewable installations in the other regions are also encouraging. For instance, the hydrogeomorphology of the Hindu Kush Himalayan is appropriate for installing small scale hydropower systems [70]-[72] - it is riparian and mountainous. Nepal, a country in that region, has high hydropower potential with proven economic feasibility [73]-[78]. Its annual average water run-off is 225 billion m3 from more than 6,000 rivers and other stream flows [79]. Therefore, local communities can greatly benefit from localised hydropower compared to other energy sources, such as portable diesel generators and solar energy.
The findings for EWS indicated that African, as well as Asian countries were in need of flood warnings. Both the Sub-Saharan [80]-[82] and Hindu Kush Himalayan [83]-[88] regions have experienced increasing vulnerability to the impacts of climate change, particularly floods, due to increased temperatures [89], [90]. Figure 3 presents the Overall Water Risk Map, developed by the World Resource Institute’s Aqueduct tool [91]. It presents evidence that Africa, Southeast Asia, Hindu Kush Himalayan and Latin America are at great risk of water-based disasters.
Overall Water Risk Map.
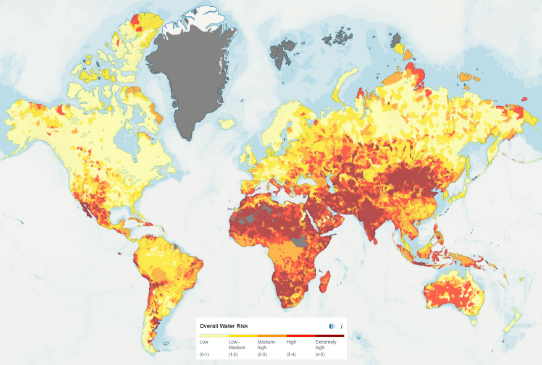
However, it should be noted that besides the identified similarities above, differences between communities were obvious and this should be highlighted. Differences in populations exist not only between different countries and regions, but also within the same communities. Therefore, any systems developed for remote communities cannot be considered as a “one size fits all” solution - it must be co-developed with community stakeholders based on their knowledge of local conditions, values and needs, and the available/needed resources to sustain these solutions over time [92], [93].
In addition, the review identified common factors that undermined or strengthened systems’ workability and longer-term use by applying the combined appropriate technology and systems evaluation tool. The findings stress the importance of collaboration between end-user communities and professionals, including key interest groups. Equity in resources and benefits, consideration of traditional or local knowledge in the processes, and community-centred management clearly contribute to increase efficiency in similar interventions [93]. A key finding with both EWS and OGRES was that community end-user groups were often minimally involved with system development, deployment and maintenance. This reflected a lack of community-focused development by implementing groups, yet it also shows community perceptions of the insufficient gain derived from them. Further, there is a clear lack of community stewardship over such systems, a critical asset in these contexts if the systems are to be maintained and scaled up over time.
The scoping review highlighted those indicators related to the complexity of the systems and unfamiliarity of end-users in proper installation, repair and maintenance. Automatic, low-tech and user-friendly localised solutions (e.g. portable, plastic, colour warning rain gauges) that correspond to the limited capabilities and resources of vulnerable communities could strengthen the success of future systems. Site-conditional indicators causing dysfunctionality in the system were also identified. As the impacts of climate change can be intense, the development of such units should endure products of extreme weather events (e.g. logs and floating debris). Climate change is contributing to more unstable weather patterns, resulting in more frequent and extreme weather events [94]. This leads to a twofold issue; it is imperative to build stronger systems in order to survive such disasters, but they must also be effective in terms of their maintenance and operations in order to overcome prolonged dry/wet periods so that they can re-focus a community’s attention when flood risk returns.
Finally, one flood EWS that was self-powered by mini solar panels was detected in the review [95]. While there was no information about how other EWS were powered, this case study can be considered as a ‘proof of concept’ hybrid system, where renewable energy is used to power the EWS alone (i.e. system-level power). Solar energy was one of the most common type of OGRES in the reviewed case studies (along with hydropower). Collectively, this evidence suggests that that a ‘scaled-up’ hybrid system is feasible and could be developed, for example, a set of panels that support ‘everyday’ energy needs could also power local EWS (for flood and other hazard types).
A potential hybrid system for renewable energy generation and flood-warning detection at the local level could have multiple benefits for vulnerable communities and their ecosystems. It could generate free, clean continuous electricity securing energy availability to local end-users. It could also detect water level changes and inform nearby communities of potential flood development, increasing awareness and response levels. However, the data gathered regarding its constituent systems indicate that a hybrid system would need to address key issues to support successful and sustainable use in these contexts, including:
The selection of appropriate OGRES type and parts. This should be decided based on local atmospheric and hydrogeomorphological conditions. For instance, solar panels for covering both EWS and community energy needs in mountainous areas, such as the Hindu Kush Himalayan region, may not be an appropriate solution. A key issue is the availability of solar energy equipment in the local area. In the case of malfunction (e.g. a broken solar panel), end-users may not be able to find replacement parts in the local markets or conduct repairs on their own, undermining the long-term feasibility of these systems. In this scenario, small scale hydropower generators may be the optimal solution for remote, riparian communities.
The selection of appropriate EWS-part type: Due to the differences in response levels between communities, the flood warnings should consist of localised sirens and outdoor lights so as to serve more populations with limited capabilities. These warning devices are widely available, energy-efficient, and easy to repair or replace. Colour code variations (e.g. traffic light colour gauges, emergency lighting or different siren tones depending on flood development stages) could prepare local communities more effectively in case of scheduled or immediate evacuation.
Potential solutions to these issues could be extrapolated from the reviewed data and inform the design and development of this system so as to meet its dual aims: supporting socio-economic development and increasing flood/hazard response capabilities. Drawing on these findings, a hybrid system should combine community-level flood risk education with training planning (e.g. evacuation drills). This would increase the response efficiency, particularly in remote communities. Based on local environmental conditions (e.g. water flow) the system should be able to generate minimum energy amounts to cover basic energy needs under both normal and extreme conditions. Excess energy could be stored in batteries for later use or delivered to other community needs.
Even when systems are seen by remote communities as providing community benefits, local participation often remains minimal. One solution to this could be a more direct and substantial involvement of communities at the planning and development stages. For example, aid organizations or civil protection authorities could work with local populations to prioritise needs, both power use and hazard management. This was the case with local communities in Nepal [14], [95]. As the system may not be able to satisfy every energy need, consultation about equitable use or common purpose may support harmonious and sustainable maintenance of the system. For example, the system could power a local classroom or public lights. Under normal conditions such lights could provide safety during the night, or increased working or study hours. This could increase income, and consequently community interest and maintenance of the system. During floods, the lights could act as emergency lights (e.g. powering an evacuation route and community hall/shelter). These developed capabilities could be used to increase disaster risk awareness and improve response (e.g. evacuation drills). This may be of particular benefit to the community’s planning for, and developing the capabilities of, vulnerable groups such as the elderly and people with mobility issues.
The co-design of such systems between professional and community stakeholders could increase community interest in the longer term. Professional stakeholders should provide information on technical aspects of the system, while community engagement should emphasise traditional know-how, and address potential concerns about changes for the community, and enhance familiarization with the system’s functions. In such instances, a system is less likely to be ‘foreign’ and different community groups are more likely to collaborate to support proper operation. This approach would minimise failures. In order to increase end-users’ involvement in pre and post-installation phases, the system could be made of readily available materials (e.g. recycled components or parts that are available in most countries and online) and/or be designed using do-it-yourself (DIY), and easy-to-deploy-and-operate (EDO) techniques [96]. These techniques increase system adaptation through community participation [97]. Such systems are widely known for their ease of assembly, deployment and capacity to be repaired (e.g. home furniture and appliances) without the need of professionals. They also include aspects of leisure, work and education, and are used across a range of fields including engineering, medicine, emergency management, energy and occupational safety [98]. Importantly, some small-scale hydropower and solar energy projects are designed based on these techniques [99].
Such approaches offer advantages to humanitarian engineering while also supporting perceived ‘ownership’ within beneficiary communities [97], and would include the potential to minimise or eliminate reliance on external technicians and promote community-based skills centred on repair and ongoing maintenance. Community access and oversight of this kind could reduce the risk of technological failures. In order to increase endurance against weather extremes and other environmental impacts, the appropriate location of systems could be determined based on hierarchical flood risk models at the local level. This data could then inform community deliberations as to placement and use, as well as contributing to the resolution of other issues to be factored in regarding site selection.
This article has critically reviewed cases of communities using OGRES and EWS. It presented existing concepts and emphasised failure and success indicators and their reported sources using a combined tool for appropriate technology and sustainability evaluation. The wider aim of this study was to consider the feasibility of an integrated system supporting both the flood resilience and socio-economic development of remote communities. The study also explored how lacking capabilities in energy generation and flood resilience affect local populations. Vulnerable communities in low and lower-middle income countries are at higher risk, and external assistance is often needed to establish suitable protections. While humanitarian engineering interventions can provide such support, it is common for these interventions to fail over time, often because they are not designed in conjunction with the community, or with specific regard to the community’s context and needs.
Recommended features for more appropriate design include: the input of community stakeholders; technology appropriateness for the consideration of local/traditional knowledge in the processes; scaling of community demands based on energy availability; and use of techniques such as the DIY and EDO in assembling and maintaining systems were highlighted.
Further research is needed to better understand how local knowledge can best adapt to these technologies in order to strengthen the feasibility analysis of hybrid systems. Such systems have the potential to support local ecosystems in sustainable ways, as well as provide vital services to remote populations.
Future studies could include databases and journals that are not considered in this scoping review. This would allow more comprehensive findings. Follow-up research should also focus on how the COVID-19 pandemic affected the sustainability of OGRES and EWS in remote communities, and how local energy and disaster response needs changed or escalated during the lockdown restrictions.
Diversification and localization of energy systems for sustainable development and energy security ,Energy Policy , Vol. 33 (17),pp 2237-2243 , 2005, https://doi.org/https://doi.org/10.1016/j.enpol.2004.05.002.
, , Divyam and Parajuli, Off-grid renewable energy solutions to expand electricity access?: An opportunity not to be missed , 2019
, On the Way of Policy Making to Reduce the Reliance of Fossil Fuels: Case Study of Iran ,Sustainability , Vol. 12 (24),pp 10606 , 2020, https://doi.org/https://doi.org/10.3390/su122410606
, A review of Africa’s transition from fossil fuels to renewable energy using circular economy principles ,Renew. Sustain. Energy Rev. , Vol. 137 ,pp 110609 , 2021, https://doi.org/https://doi.org/10.1016/j.rser.2020.110609
, - , List of lower and lower middle-income countries, World Bank Country and Lending Groups
OFF-GRID RENEWABLE ENERGY SOLUTIONS Global and regional status and trends ,International Renewable Energy Agency (IRENA) , 2018
, PV-Hybrid Off-Grid and Mini-Grid Systems for Rural Electrification in Sub-Saharan Africa ,Smart Grid Renew. Energy , Vol. 09 (10),pp 171-185 , 2018, https://doi.org/https://doi.org/10.4236/sgre.2018.910011
, Transforming our world: The 2030 agenda for sustainable development , 2016
, Heat, light and power for refugees: saving lives, reducing costs , 2015
, Challenges and opportunities of new energy schemes for food security in humanitarian contexts: A selective review ,Sustain. Energy Technol. Assessments , Vol. 22 ,pp 208-219 , 2017, https://doi.org/https://doi.org/10.1016/j.seta.2017.02.006
, The UN Sendai Framework for Disaster Risk Reduction 2015-2030: Negotiation Process and Prospects for Science and Practice ,J. Extrem. Events , Vol. 02 (01),pp 1571001 , 2015, https://doi.org/https://doi.org/10.1142/s2345737615710013
, Links between natural disasters, humanitarian assistance and disaster risk reduction: A critical perspective ,Occas. Pap. , Vol. 15 , 2007
, Financial instruments for disaster risk management and climate change adaptation ,Clim. Change , Vol. 133 (1),pp 85-100 , 2015, https://doi.org/https://doi.org/10.1007/s10584-013-1035-6
, Socio-economic impacts of a micro-hydropower plant on rural livelihoods ,Sci. Res. Essays , Vol. 6 (19),pp 3964-3972 , 2011, https://doi.org/https://doi.org/10.5897/sre10.766
, Early warning systems and livelihood resilience: Exploring opportunities for community participation ,UNU-EHS Work. Pap. Ser. , Vol. 1 , 2014
, Sub-Saharan Africa makes progress against poverty but has long way to go ,Pew Research Center , 2015
, Energy Access and Electricity Planning ,State Electr. access Rep. ,pp 16 , 2017
, Combining Energy Efficiency and Disaster Mitigation Efforts in Residential Properties ,Office of Policy Development and Research , 2017
, Humanitarian engineering and vulnerable communities: hydropower applications in localised flood response and sustainable development ,Int. J. Sustain. Energy , Vol. 39 (10),pp 941-950 , 2020, https://doi.org/https://doi.org/10.1080/14786451.2020.1779274
, Humanitarian engineering at the Colorado school of mines: An example of multidisciplinary engineering ,in ASEE Annual Conference Proceedings ,pp 10161-10175 , 2003, https://doi.org/https://doi.org/10.18260/1-2--11871
, What do students understand by the term ‘Humanitarian Engineering’? , 2012
, Humanitarian Engineering: A New Interdisciplinary Course on the Application of Engineering Skills to Local and Global Humanitarian Challenges , 2018, https://doi.org/https://doi.org/10.24908/pceea.v0i0.12963
, Dare to prepare: taking risk seriousl y Summary ,pp 1-18 , 2014
, Five Guiding Principles to Enhance Community Participation in Humanitarian Engineering Projects. ,J. Humanit. Eng. , Vol. 5 (2), 2017, https://doi.org/https://doi.org/10.36479/jhe.v5i2.80
, Sustainable humanitarian engineering in practice - The East Bali Poverty Project ,Proc. Inst. Civ. Eng. Munic. Eng. , Vol. 171 (2),pp 67-77 , 2018, https://doi.org/https://doi.org/10.1680/jmuen.16.00034
, Community Empowerment through Appropriate Technology: Sustaining the Sustainable Development ,Procedia Environ. Sci. , Vol. 17 ,pp 1007-1016 , 2013, https://doi.org/https://doi.org/10.1016/j.proenv.2013.02.120
, Interface between appropriate technology and sustainable energy policy in vulnerable societies ,Sustain. Cities Soc. , Vol. 12 ,pp 9-15 , 2014, https://doi.org/https://doi.org/10.1016/j.scs.2013.10.003
, Technological solution for vulnerable communities: How does its approach matter? ,IOP Conf. Ser. Mater. Sci. Eng. , Vol. 58 ,pp 012022 , 2014, https://doi.org/https://doi.org/10.1088/1757-899X/58/1/012022
, Technological Solution for Vulnerable Communities: Reverse Engineering Below the Radar ,Adv. Sci. Lett. , Vol. 20 (10),pp 2282-2286 , 2014, https://doi.org/https://doi.org/10.1166/asl.2014.5726
, Sustainability assessment of renewable energy projects for off-grid rural electrification: The Pangan-an Island case in the Philippines ,Renew. Sustain. Energy Rev. , Vol. 16 (1),pp 54-64 , 2012, https://doi.org/https://doi.org/10.1016/j.rser.2011.07.136
, How effective are small-scale energy interventions in developing countries? Results from a post-evaluation on project-level ,Appl. Energy , Vol. 135 ,pp 809-814 , 2014, https://doi.org/https://doi.org/10.1016/j.apenergy.2014.05.032
, Quantitative assessment of appropriate technology ,Procedia Eng. , Vol. 78 ,pp 345-358 , 2014, https://doi.org/https://doi.org/10.1016/j.proeng.2014.07.076
, Indicators for assessment of rural electrification-An approach for the comparison of apples and pears ,Energy Policy , Vol. 36 (7),pp 2665-2673 , 2008, https://doi.org/https://doi.org/10.1016/j.enpol.2008.03.023
, And then they lived sustainably ever after?-Assessment of rural electrification cases by means of indicators ,Energy Policy , Vol. 36 (7),pp 2674-2684 , 2008, https://doi.org/https://doi.org/10.1016/j.enpol.2008.03.022
, Scoping studies: Towards a methodological framework ,Int. J. Soc. Res. Methodol. Theory Pract. , Vol. 8 (1),pp 19-32 , 2005, https://doi.org/https://doi.org/10.1080/1364557032000119616
, GPS water level measurements for Indonesia’s tsunami early warning system ,Nat. Hazards Earth Syst. Sci. , Vol. 11 (3),pp 741-749 , 2011, https://doi.org/https://doi.org/10.5194/nhess-11-741-2011
, Design and implementation of a landslide early warning system ,Eng. Geol. , Vol. 147-148 ,pp 124-136 , 2012, https://doi.org/https://doi.org/10.1016/j.enggeo.2012.07.017
, SMS based flood monitoring and early warning system ,ARPN J. Eng. Appl. Sci. , Vol. 10 (15),pp 6387-6391 , 2015
, Humanitarian engineering at the sustainability-development nexus: mapping vulnerability and capability factors for communities at risk of water-based disasters ,Sustain. Sci. , 2021, https://doi.org/https://doi.org/10.1007/s11625-020-00890-y
, A scoping review of scoping reviews: Advancing the approach and enhancing the consistency ,Res. Synth. Methods , Vol. 5 (4),pp 371-385 , 2014, https://doi.org/https://doi.org/10.1002/jrsm.1123
, Women and WASH in Nepal: a scoping review of existing literature ,Water Int. , Vol. 45 (3),pp 222-245 , 2020, https://doi.org/https://doi.org/10.1080/02508060.2020.1754564
, Precarity and Aging: A Scoping Review ,Gerontologist , Vol. 60 (8),pp e620-e632 , 2020, https://doi.org/https://doi.org/10.1093/geront/gnz135
, Older Adults’ Self-Perceptions of Aging and Being Older: A Scoping Review ,Gerontologist , Vol. 60 (7),pp e524-e534 , 2020, https://doi.org/https://doi.org/10.1093/geront/gnz153
, Online university education is the new normal: but is face-to-face better? ,Interact. Technol. Smart Educ. , Vol. ahead-of-p (ahead-of-print), 2021, https://doi.org/https://doi.org/10.1108/ITSE-08-2020-0181
, Battery hazards and safety: A scoping review for lead acid and silver-zinc batteries ,Saf. Sci. , Vol. 140 ,pp 105290 , 2021, https://doi.org/https://doi.org/10.1016/j.ssci.2021.105290
, Micro-hydropower impact on communities’ livelihood analysed with the capability approach ,Energy Sustain. Dev. , Vol. 45 ,pp 206-210 , 2018, https://doi.org/https://doi.org/10.1016/j.esd.2018.07.003
, A Study of Socioeconomic Impacts of Renewable Energy Projects in Afghanistan ,Procedia Eng. , Vol. 145 ,pp 995-1003 , 2016, https://doi.org/https://doi.org/10.1016/j.proeng.2016.04.129
, Using thematic analysis in psychology. ,Qual. Res. Psychol. , Vol. 3 (2),pp 77-101 , 2006
, The economics of renewable energy expansion in rural Sub-Saharan Africa ,Energy Policy , Vol. 39 (1),pp 215-227 , 2011, https://doi.org/https://doi.org/10.1016/j.enpol.2010.09.034
, Drivers and barriers to rural electrification in Tanzania and Mozambique - Grid-extension, off-grid, and renewable energy technologies ,Renew. Energy , Vol. 61 ,pp 117-124 , 2014, https://doi.org/https://doi.org/10.1016/j.renene.2012.09.057
, Adoption rationales and effects of off-grid renewable energy access for African youth: A case study from Tanzania ,Renew. Sustain. Energy Rev. , Vol. 141 ,pp 110793 , 2021, https://doi.org/https://doi.org/10.1016/j.rser.2021.110793
, Benefits and challenges to productive use of off-grid rural electrification: The case of mini-hydropower in Bulongwa-Tanzania ,Energy Sustain. Dev. , Vol. 53 ,pp 97-103 , 2019, https://doi.org/https://doi.org/10.1016/j.esd.2019.10.001
, Towards sustainable financing models for micro-hydro plants in Sub-Saharan African countries: A theoretical review ,J. Econ. Int. Financ. , Vol. 8 (3),pp 19-33 , 2016, https://doi.org/https://doi.org/10.5897/jeif2016.0750
, The empirical reality & sustainable management failures of renewable energy projects in Sub-Saharan Africa (part 1 of 2) ,Renew. Energy , Vol. 102 ,pp 234-240 , 2017, https://doi.org/https://doi.org/10.1016/j.renene.2016.10.037
, Status of renewable energy consumption and developmental challenges in Sub-Sahara Africa ,Renew. Sustain. Energy Rev. , Vol. 27 ,pp 453-463 , 2013, https://doi.org/https://doi.org/10.1016/j.rser.2013.06.044
, Economic performance, environmental concerns, and renewable energy consumption: drivers of renewable energy development in Sub-Sahara Africa ,Clean Technol. Environ. Policy , Vol. 19 (2),pp 437-450 , 2017, https://doi.org/https://doi.org/10.1007/s10098-016-1229-5
, A community based approach for sustainable off-grid PV systems in developing countries ,in 2011 IEEE Power and Energy Society General Meeting ,pp 1-7 , 2011, https://doi.org/https://doi.org/10.1109/PES.2011.6039593
, Off-grid rural electrification planning in Sub-Saharan Africa using renewable energy systems: the case of photovoltaics in the Republic of Djibouti ,
, Optimization and cost-benefit assessment of hybrid power systems for off-grid rural electrification in Ethiopia ,Energy , Vol. 177 ,pp 234-246 , 2019, https://doi.org/https://doi.org/10.1016/j.energy.2019.04.095
, Potential of Small-Scale Hydropower for Electricity Generation in Sub-Saharan Africa ,ISRN Renew. Energy , Vol. 2012 ,pp 1-15 , 2012, https://doi.org/https://doi.org/10.5402/2012/132606
, Solar Photovoltaics in Sub-Saharan Africa - Addressing Barriers, Unlocking Potential ,Energy Procedia , Vol. 106 ,pp 97-110 , 2016, https://doi.org/https://doi.org/10.1016/j.egypro.2016.12.108
, Financing energy access and off-grid electrification: A review of status, options and challenges ,Renew. Sustain. Energy Rev. , Vol. 20 ,pp 462-472 , 2013, https://doi.org/https://doi.org/10.1016/j.rser.2012.12.008
, Barriers and drivers to renewable energy investment in sub-Saharan Africa ,J. Environ. Invest. , Vol. 2 (1),pp 54-80 , 2011
, Issues and applications of real-time data from off-grid electrical systems ,in 2016 IEEE PES PowerAfrica ,pp 88-92 , 2016, https://doi.org/https://doi.org/10.1109/PowerAfrica.2016.7556577
, Lighting and Electricity Services for Off-Grid Populations in Sub-Sahara Africa , 2018
, Energy (in)justice in off-grid rural electrification policy: South Africa in focus ,Energy Res. Soc. Sci. , Vol. 44 ,pp 152-171 , 2018, https://doi.org/https://doi.org/10.1016/j.erss.2018.05.002
, Off-grid solar PV: Is it an affordable or appropriate solution for rural electrification in Sub-Saharan African countries? ,Renew. Sustain. Energy Rev. , Vol. 60 ,pp 1405-1418 , 2016, https://doi.org/https://doi.org/10.1016/j.rser.2016.03.016
, The troubled path to ending darkness: Energy injustice encounters in Malawi’s off-grid solar market ,Energy Res. Soc. Sci. , Vol. 69 ,pp 101712 , 2020, https://doi.org/https://doi.org/10.1016/j.erss.2020.101712
, SDG7: Data and Projections ,SDG7: Data and Projections , 2020
, - , , in The Hindu Kush Himalaya Assessment, 2019
Hydropower development in the Hindu Kush Himalayan region: Issues, policies and opportunities ,Renew. Sustain. Energy Rev. , Vol. 107 ,pp 446-461 , 2019, https://doi.org/https://doi.org/10.1016/j.rser.2019.03.010
, Water and hydropower in the green economy and sustainable development of the Hindu Kush--Himalayan region ,J. Int. Assoc. Electr. Gener. Transm. Distrib. , Vol. 25 (2),pp 21-30 , 2012
, Promotional issues on alternative energy technologies in Nepal ,Energy Policy , Vol. 31 (4),pp 307-318 , 2003, https://doi.org/https://doi.org/10.1016/S0301-4215(02)00043-5
, Hydropower development in Nepal ,NRB Econ. Rev. , Vol. 18 ,pp 70-94 , 2006
, A Review of Hydropower Projects in Nepal ,Energy Procedia , Vol. 110 ,pp 581-585 , 2017, https://doi.org/https://doi.org/10.1016/j.egypro.2017.03.188
, Hydropower development and economic growth in Nepal , 2020
, Hydropower development in Nepal ,Renew. Sustain. Energy Rev. , Vol. 21 ,pp 684-693 , 2013, https://doi.org/https://doi.org/10.1016/j.rser.2013.01.013
, Current status of renewable energy in Nepal: Opportunities and challenges ,Renew. Sustain. Energy Rev. , Vol. 15 (8),pp 4107-4117 , 2011, https://doi.org/https://doi.org/10.1016/j.rser.2011.07.022
, Hydropower Development and Its Sustainability With Respect to Sedimentation in Nepal ,J. Inst. Eng. , Vol. 7 (1),pp 56-64 , 1970, https://doi.org/https://doi.org/10.3126/jie.v7i1.2063
, Impact of Floods on Livelihoods and Vulnerability of Natural Resource Dependent Communities in Northern Ghana ,Water , Vol. 2 (2),pp 120-139 , 2010, https://doi.org/https://doi.org/10.3390/w2020120
, Emerging issues in the management of floods in ghana ,Int. J. Saf. Secur. Eng. , Vol. 1 (2),pp 182-192 , 2011, https://doi.org/https://doi.org/10.2495/SAFE-V1-N2-182-192
, Effects of floods on infrastructure users in Kenya ,J. Flood Risk Manag. , 2021, https://doi.org/https://doi.org/10.1111/jfr3.12746
, Managing flash floods and sustainable development in the Himalayas , 2006
, Gender and Disaster Resilience in the Hindu Kush Himalayan Region ,pp 233-249 , 2015
, Mapping the vulnerability hotspots over Hindu-Kush Himalaya region to flooding disasters ,Weather Clim. Extrem. , Vol. 8 ,pp 46-58 , 2015, https://doi.org/https://doi.org/10.1016/j.wace.2014.12.001
, - , , in The Hindu Kush Himalaya Assessment, 2019
How does climate change affect human health in the Hindu Kush-Himalaya region ,in Regional Health Forum, 2008 , Vol. 12 (1),pp 11-15 ,
, Floods in the Hindu Kush Region: Causes and Socio-Economic Aspects ,pp 33-52 , 2015
, The Niger , a lifeline: effective water management in the Upper Niger Basin ,Africa (Lond) , (Ivm),pp 304 , 2005
, , United Nations Framework Convention on Climate Change , 2020
, Overall Water Risk ,Water Risk Atlas ,
, Enhancing Research Impact in International Development: A Practical Guide for Practitioners and Researchers ,2020 ,
, Using off-grid hydropower for community-led flood resilience: an integrated systems approach ,Int. J. Sustain. Energy ,pp 1-15 , 2021, https://doi.org/https://doi.org/10.1080/14786451.2021.1961773
, IPCC Special Report on Managing the Risks of Extreme Events and Disasters to Advance Climate Change Adaptation (SREX) ,J. Epidemiol. Community Health , Vol. 66 (9),pp 759-760 , 2012, https://doi.org/https://doi.org/10.1136/jech-2012-201045
, Community-based Flood Early Warning System: The Story from Then to Now ,2018 ,
, Design of a do-it-yourself (DIY) based solar-powered led lighting system for training and empowering rural youth , 2019
, Advancing Alternative Pathways to Science: Community Partnership, Do-It-Yourself (DIY)/Do-It-Together (DIT) Collaboration, and STEM Learning ‘from Below,’ ,Transform. J. Incl. Scholarsh. Pedagog. , Vol. 27 (1),pp 41-50 , 2017, https://doi.org/https://doi.org/10.1353/tnf.2017.0004
, DIY solar projects ,How to put the sun to work in your home , 2011
, Electricity autonomy and power grids in Africa: From rural experiments to urban hybridizations ,in Local Energy Autonomy: Spaces, Scales, Politics ,pp 291-314 , 2019
, Understanding sustainable operation of micro-hydropower: a field study in Nepal ,Energy Sustain. Dev. , Vol. 57 ,pp 12-21 , 2020, https://doi.org/https://doi.org/10.1016/j.esd.2020.04.007
, Opportunities and challenges to rural renewable energy projects in Africa: Lessons from the Esaghem Village, Cameroon solar electrification project ,Renew. Energy , Vol. 131 ,pp 1013-1021 , 2019, https://doi.org/https://doi.org/10.1016/j.renene.2018.07.092
, A socio-technical approach to increasing the battery lifetime of off-grid photovoltaic systems applied to a case study in Rwanda ,Renew. Energy , Vol. 83 ,pp 30-40 , 2015, https://doi.org/https://doi.org/10.1016/j.renene.2015.04.020
, Addressing the current remote area electrification problems with solar and microhydro systems in Central Africa ,Renew. Energy , Vol. 67 ,pp 10-19 , 2014, https://doi.org/https://doi.org/10.1016/j.renene.2013.11.044
, Identification of stakeholders for sustainable renewable energy applications in Cameroon ,Renew. Sustain. Energy Rev. , Vol. 16 (7),pp 4661-4666 , 2012, https://doi.org/https://doi.org/10.1016/j.rser.2012.05.019
, The socio-technical barriers to Solar Home Systems (SHS) in Papua New Guinea: ‘Choosing pigs, prostitutes, and poker chips over panels,’ ,Energy Policy , Vol. 39 (3),pp 1532-1542 , 2011, https://doi.org/https://doi.org/10.1016/j.enpol.2010.12.027
, Lessons from flood early warning systems ,Environ. Sci. Policy , Vol. 58 ,pp 117-122 , 2016, https://doi.org/https://doi.org/10.1016/j.envsci.2016.01.006
,